Nanostructured electrodes for simultaneous power and energy
The group pursues 3D electrode structures for batteries because thin storage layers allow fast ion transport and thereby achieve high power at high energy. On the other hand, if storage layers are thin, they must occupy large surface area so that energy density is not compromised. This constraint leads naturally to 3-D architectures of materials that provide ion access from electrolyte to storage material over large areas.
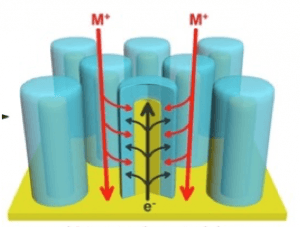
Nanoelectrodes for high power and energy can be achieved by providing ion and electron transport paths intimately connected
Since most ion storage materials are not good electronic conductors (at least at some level of ion loading), it is typically important to provide electron current collecting structures intimately contacting thin ion storage layers. In this way transport of ions from one electrode to the other can be rapidly charge balanced by the fast electron transport. The resulting heterogeneous 3-D structures avoid problems associated with low electronic conductivity of electrode materials as well as their changing conductivity with ion concentration.
Design and fabrication of dense mesoscale architectures
Nanostructured batteries must directly address the structure and architecture issues associated with this constraint in the design of individual nanostructures and of a cell where 3D nanostructures are densely packed together to retain high energy density (gravimetric, volumetric, or areal). Finally, the structures must be built to accommodate sometimes significant volume and phase change of the active ion storage material as ion insertion and deinsertion takes place as a consequence of charge/discharge cycling of the battery.
Favorable designs to accomplish this are indeed challenging, from initial design and modeling that scales relative dimensions suitably, to creation of an appropriate scaffold on which to synthesize the active layers, to wiring and contacting massive arrays. Furthermore, these strategies must be realized in materials synthesis and workable within the limitations of process equipment suitable for the task. In a sense this poses complexity of device, process, and equipment similar to that in the semiconductor chip domain.
Examples of 3-D architectures
Nanobattery sponge electrodes present an embodiment of nanostructured electrodes integrating electron current collectors with conformal ion storage materials covering them. Starting with carbon nanotubes (CNT) rather randomly assembled as an electron-conducting sponge, ALD electrode materials were deposited on the CNT surfaces. Together the ALD-CNT combination provides a nanostructured electrode architecture which we deem “pseudo-random”. While the originating CNT sponge had a long-range random configuration, conformal deposition of ion storage material produced well-defined and identical short-range structure along the CNT’s.
As a precursor to our NEES EFRC, we demonstrated the benefits of high surface area 3-D arrays for energy storage in an electrostatic nanocapacitor array, formed by creating a nanoporous anodic aluminum oxide (AAO) template, then conformally depositing by ALD metal, dielectric, and metal layers over the deep AAO, thus achieving re
cord areal capacitance density. The benefits of this architecture have been widely recognized for electronics applications. The templating method provides a clear example of “regular” architectures, in which the nanostructures are not only essentially identical, but display some degree of longer-range ordering.
As a follow-up we subsequently developed and demonstrated nanopore batteries formed from a similar AAO template. Here ALD current collectors and then ALD electrodes were created at each end of the pores, then liquid organic electrolyte was filled into the pores to complete the high density array of nanobatteries. Results confirm the benefits of designs integrating large area thin ion storage layers intimately connected to electron transport layers.
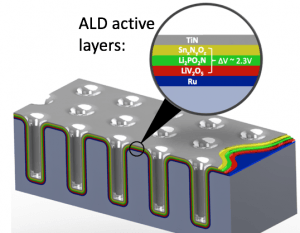
3D solid state battery synthesized by conformal ALD deposition of cathode, solid electrolyte, anode, and current collecting layers into etched trenches in Si
Most recently we created 3-D solid state battery arrays with interdigitated electrodes and a solid electrolyte (LiPON), using microscale pores etched into Si wafers and a 5-layer ALD process sequence for current collectors, anode and cathode layers, and electrolyte between anode and cathode.
Role of thin film process technology
Our research in energy storage is primarily based on thin film process technology as a synthesis strategy. In general, thin film technology includes processes for materials deposition, etching, and patterning that enable a wide variety of 3-D micro- and nano-structures to be created as designed. These approaches are central to semiconductor electronic chips, displays, information storage, optoelectronics, and other technology arenas.
In contrast, the overwhelming plurality of batteries are manufactured very differently, using electrode particles of differing size/shape to make composite electrodes with varying density and porosity. Why then do we pursue battery research using thin film technology?
First, by creating model structures – individual electrode nanostructures, full nanostructured electrodes in a conventional battery, and fully interdigitated electrodes in an all-solid-state battery, we believe we can much better reveal the science underlying electrochemical storage. Indeed that is the goal of our NEES EFRC program.
Second, we can simultaneously explore the possibilities that controlled thin film battery structures might offer as a disruptive battery technology. Specialized applications have stimulated a thin film battery industry typically producing planar batteries by physical vapor deposition of single anode, solid electrolyte, and cathode, with associated current collectors. Our research asks not only how well such layers and interfaces can be controlled and what performance results, but also how might those materials be arranged as 3-D structures and mesoscale architectures to harvest the benefits of 3-D structuring we have seen in liquid electrolyte batteries.